
Pigs
Counteracting strategies
Due to the fact that preventive methods during crop growth, harvesting and storage only reduce the potential risk of mycotoxin contamination, detoxification procedures after harvest are necessary, these processes should:- deactivate, destroy or remove the toxin
- not result in the deposition of toxic substances, metabolites or toxic byproducts in the feed
- retain nutrient value and acceptability of the feed through the animal
- not result in significant alterations in the product's technological properties
- destroy the fungal spores
Furthermore, the process should be readily available, easily utilized, inexpensive and the effects on the environment should also be considered. Detoxification procedures are divided into three categories: physical, chemical, biological methods (adsorption and biotransformation).
» Physical Methods | » Chemical Methods |
Cleaning | |
Mechanical sorting and separation | » Biological Methods |
Washing | Adsorption |
Density segregation | Biotransformation |
Thermal inactivation |
Physical methods
CleaningDuring the cleaning process of contaminated grain dust, husks, hair and shallow particles are blown away by aspiration or scouring.
Mechanical sorting and separation
In this process the clean product is separated from mycotoxin-contaminated grains. High feed losses are possible due to incomplete and uncertain separation. Therefore mechanical sorting and separation is not cost-efficient.
Washing
Washing procedures using water or sodium carbonate solution result in some reduction of mycotoxins in grains.
Density segregation
For the segregation of contaminated grain flotation can be used. This method can reduce mycotoxin contamination but it should be noted, that appearance and weight of a particular kernel does not necessarily indicate mycotoxin contamination.
Thermal inactivation
Mycotoxins are very heat stable therefore heat treatments like boiling water, roasting or even autoclaving cannot adequately destroy them.
Other physical methods are irradiation (including microwaves), ultrasound or solvent extraction.
However, the efficacy of physical treatments depends on the level of contamination and the distribution of mycotoxins throughout the grain. Additionally the results obtained are often uncertain and connected with high losses. Furthermore these methods are often very expensive. Thus the practical application of physical treatment is very limited.
Chemical methods
Various chemicals (bases, oxidizing agents, different gases …) have been tested for detoxification of mycotoxins but only a limited number of chemical methods are effective against mycotoxins without reducing nutritive value and palatability of the feed. For achieving adequate decontamination results several parameters like reaction time, temperature and moisture have to be monitored. Chemical methods also need additional cleaning treatments and are therefore very expensive and time consuming. Furthermore toxic by-products may be produced. Treatment of contaminated feed with ammonia was the most common method in the past. Although early studies showed this technique to be safe and effective, ammoniation has not been approved by the US Food and Drug Administration due to the potential toxicity and carcinogenicity of the reaction product.Biological Methods
AdsorptionThe use of adsorbent materials is a very common method employed to prevent mycotoxicoses in particular aflatoxicosis. These compounds are added to the feed to bind the toxin during the digestive process in the gastrointestinal tract resulting in a reduction of toxin bioavailability. The adsorption of mycotoxins requires polarity and also a suitable position of the functional groups. Due to this fact only a few mycotoxins can be adsorbed efficiently without affecting essential feed ingredients.
Substances scientifically investigated as potential mycotoxin-binding agents include, among others:
- bentonites
- zeolites
- Diatomaceous earth
- activated charcoal
- fibers from plant sources
Mycotoxin binding is obtained with a combination of physical adsorption or physisorption (relatively weak bonding involving van der Waals interactions and hydrogen bonding) or with chemical adsorption also called chemisorption (stronger interaction which involves ionic or covalent bonding).
The critical parameters for evaluation of an applicable mycotoxin binder are:
- Chemisorption which indicates strong binding
- High adsorption capacity (g toxin/kg binder)
- High mycotoxin affinity
- No or low desorption of the already adsorbed mycotoxin
- No or low adsorption of essential nutrients
- Absence of toxicity of the binding material itself (heavy metals, dioxine…)
- Low effective inclusion rate in feed
- Rapid and uniform dispersion in the feed during mixing
- Heat stability during pelleting, extrusion and storage
- High stability over a wide pH range
In several studies, hydrated sodium calcium aluminosilicates (HSCAS) have proven to be the most promising adsorbents. However, while high-quality and scientifically explained results were obtained for counteracting aflatoxins adsorption of other mycotoxins was limited (e.g. zearalenone, ochratoxin A, fumonisin) or even failed (e.g. trichothecenes, like deoxynivalenol) under field conditions.
A widespread range of mycotoxins can be adsorbed by activated charcoal but the efficacy in feeding trials was very low. Moreover, it is an unspecific adsorbent and also binds essential nutrients.
Bentonites are clay minerals which result from the decomposition of volcanic ash and consist mainly of the phylosilicate mineral montmorillonite (smectite). Bentonites have been reported to effectively diminish the inhibitory effects of dietary aflatoxin B1.
Biotransformation
Biological detoxification of mycotoxins by enzymes and/or microorganisms, which has been researched for over thirty years, comprises of the degradation of mycotoxins within the gastrointestinal tract, before resorption into the animal occurs. This method offers a very specific, irreversible, efficient and environmentally friendly way of detoxification and leads to neither toxic residues nor any undesired byproducts. Most of these studies used mainly two biological habitats, namely rumen fluid and soil, others utilized feces of different animal species. Until now, a respectable number of microorganisms have been known to be able to counteract different mycotoxins (see table).
Biological detoxification of mycotoxins
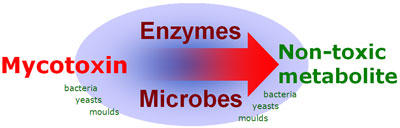
In the case of trichothecene, the 12,13-epoxide ring is mainly responsible for their toxicity. Several authors described this de-epoxidation reaction of ruminal or intestinal flora, but Binder et al. were the first to isolate a pure bacterial strain-, which was able to biotransform the epoxide group of trichothecenes. This Eubacterium (BBSH 797) strain was isolated out of bovine rumen fluid. The mode of action was proven in vitro and also in vivo by applying trichothecenes. The detoxifying strain Eubacterium BBSH 797 was the first microbe used in a mycotoxin deactivating feed additive. Furthermore a novel yeast strain, capable of degrading ochratoxin A (OTA) and zearalenone (ZON) was isolated and characterized. The strain named Trichosporon mycotoxinivorans (MTV) -detoxifies OTA by cleavage of the phenylalanine moiety from the isocumarin derivate to ochratoxin A. This metabolite has been described to be nontoxic or at least 500 times less toxic than the parent compound. Zearalenone has no acute toxicity, but shows estrogenic effects and therefore causes substantial fertility problems. The metabolisation of zearalenone by T. myctoxinivorans leads to a compound that is no longer estrogenic, which was proven in an in vitro assay using breast cancer cells.
Biotransformation (de-epoxidation) of a trichothecene into a non-toxic metabolite by de-epoxidases produced by BBSH 797
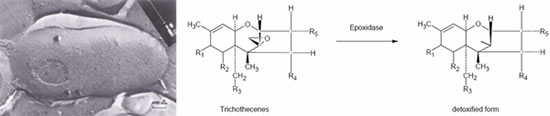
Biotransformation of ochratoxin A into a non-toxic metabolite by specific enzymes produced by T. mycotoxinivorans
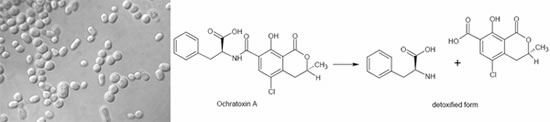
Biotransformation of zearalenone into a non-toxic metabolite by specific enzymes produced by T. mycotoxinivorans
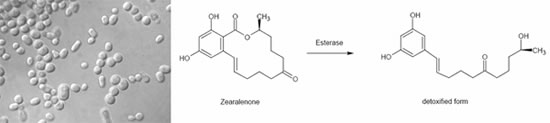
Biotransformation of mycotoxins by certain isolated microorganisms
Microorganism | Investigated mycotoxins | References |
Flavobacterium aurantiacum | Aflatoxins | Ciegler et al., 1966 |
Aspergillus flavus | Hamid and Smith, 1987 | |
Gliocladium roseum NRRL 1859 | Zearalenone | El-Sharkawy and Hajj, 1988 |
Rhodococcus erythropolis Norcardia globulera |
Rood and Duvick, 1998 | |
Saccharomyces cerevisiae | Patulin | Stinson et al., 1978 |
Paecilomyces sp | Anderson et al., 1979 | |
Phenylobaterium immobile | Ochtratoxin A | Wegst and Lingens, 1983 |
Acinetobacter calcoaceticus | Hwang and Draughon, 1994 | |
Trichosporon mycotoxinivorans (MTV) | Schatzmayr et al., 2006 | |
Exophiala pinifera | Fumonisins | Duvick et al., 1998 |
Rhinocladiella atrovirens | ||
Bacterium ATCC 55552 | ||
Agrobacterium sp. | Deoxynivalenol | Shima et al., 1997 |
Eubacterium sp. | Binder et al., 1998 | |
Butyrvibrio fibrisolvens | T-2 toxin | Westlake et al., 1987 |
Selenomonas ruminantium | ||
Anaerovibrio lipolytica | T-2 toxin | Westlake et al., 1987 |
Curtobacterium sp. | T-2 toxin | Ueno et al., 1983 |
Trichosporon mycotoxinivorans (MTV) | Zearalenone | Schatzmayr et al., 2006 |